Research Topics |
|
Plasmodesmata & the Supracellular Nature
of Plants
During the course of evolution, biological systems developed ever-increasingly
sophisticated control systems to regulate the complex processes
underlying cellular, developmental and physiological programs.
Cellular specialization, reflected throughout the animal and plant
kingdoms, required the development of a matrix of signaling pathways
with inherent plasticity to provide the organism with the capacity
to respond to a wide range of inputs.
The resultant signaling systems can be divided into two categories.
The first involves signaling agents whose actions are confined
to the individual cells in which the specific signaling process
was initiated; i.e., these molecules are cell-autonomous in behavior.
The second group involves signals that act beyond their sites of
production; i.e., these signaling agents act in a non-cell-autonomous
manner and can involve hormones, metabolites, peptides and macromolecules.
Such signals can move either via the extra-cellular pathway, which
is common to all organisms, or, in the plant kingdom, they can
traffic from cell to cell through specialized structures termed
plasmodesmata (PD).
The architecture of the PD is such that non-cell-autonomous signals
can move between neighboring cells without the need to enter the
(hostile) extracellular milieu. Recent studies have demonstrated
that PD can potentiate the cell-to-cell trafficking of proteins,
such as transcription factors, and messenger and small RNA molecules.
In addition, with the evolution of the unique vascular system of
plants, PD provide a pathway for the exchange of molecules within
the body of the plant. In view of these findings, it is thought that
PD likely played an important role in plant evolution.
|
|
Dual Modes of PD Operation
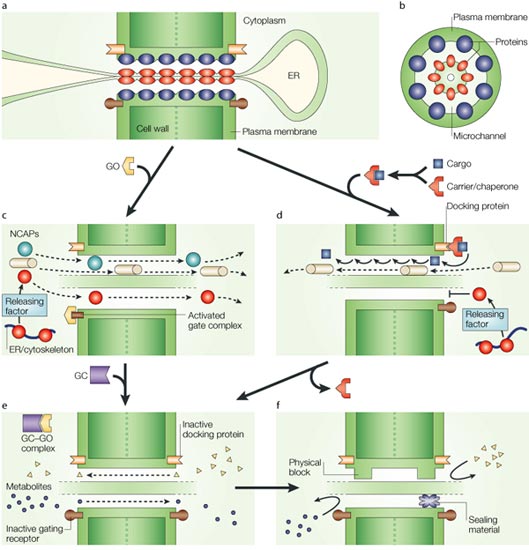
|
Plasmodesmal
architecture and potential mechanisms for cell-to-cell trafficking
of molecules. Diagrammatic illustration of PD, in longitudinal
(A) & cross-section (B) showing the
arrangement of the cytoplasmic channel formed by the continuity
of the plasma membrane & ER across the adjoining cell walls.
Proteins divide this channel into microchannels through which molecules
can pass. (C) Gating model for NCAP cell-to-cell movement. Microchannels
are shown being dilated by a gate open protein (GO) binding to
its cognate PD gating receptor. Molecules free to move within the
cytoplasm, & whose size will permit passage through dilated
microchannels, diffuse into neighboring cells; i.e., this route
functions as a non-targeted mode of movement. (D) Selective NCAP
pathway. Carriers &/or chaperones deliver cargo (protein or
ororbinding deliver cargo (protein or RNA–protein complex) to a
PD docking site that then induces microchannel dilation, followed
by selective cargo trafficking into neighboring cells. Small molecules
(metabolites or polypeptides) can co-diffuse through this dilated
microchannel. (E) Microchannel closure occurs by either removal
of GO, through an interaction with a putative gate closure protein
(GC), or carrier release from the PD docking protein. In this closed
state, NCAPs cannot move but metabolites can still be exchanged
by diffusion. (F) Symplasmic isolation of cells can
be achieved by occlusion/blockage of PD microchannels. |
|
Although these general concepts are founded on experimental
evidence, details concerning the individual components of this signaling
pathway, as well as the manner in which trafficking of non-cell-autonomous
signals might be regulated, remain sparse. Recent studies from our
laboratory have led to the development of a biochemical protocol
that allows the identification of PD components likely involved in
cell-to-cell trafficking of non-cell-autonomous proteins (NCAPs).
The first gene identified using this approach was the tobacco NON-CELL-AUTONOMOUS
PATHWAY PROTEIN 1 (NCAPP1). |
|
Role of NCAPP1 in Protein Trafficking cell
to cell |
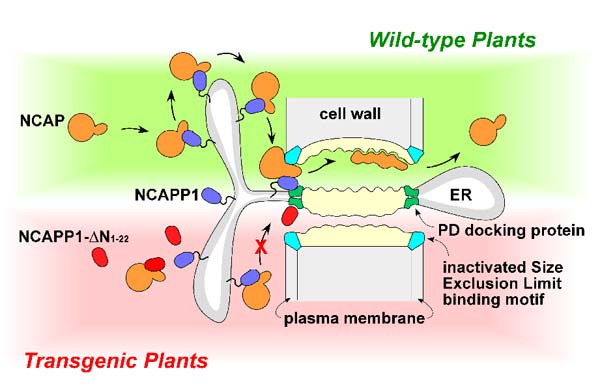 |
Model illustrating a possible mechanisms by
which NCAPP1 could be involved in mediating delivery of NCAPs to
PD. Blockage of this pathway, in transgenic NCAPP1-ΔN 1-22 plants,
may well reflect an inability of this mutant form of NCAPP1 to
be anchored to the ER. The formation of a dysfunctional complex
could then block access to putative PD docking sites (red shading)
(see Lee et al. 2003). |
|
A major goal of our lab is to
identify further components of the PD supramolecular complex and
regulators of NCAP trafficking/PD function. For these studies
we are using a range of experimental approaches, including mutant
screening, symplasmic domain mapping via confocal microscopy, candidate
protein purification and characterization using biochemical methods,
protein-protein interaction (via FRET analysis), gene silencing,
etc. Knowledge gained on the molecular aspects of the NCAP pathway
will allow us to more fully understand the hierarchy of controls
that operate at the cellular, tissue and whole-plant levels. |
|
Role for PD in Delivery of Systemically
Acting NCAPs |
|
Phloem in mature leaves functions as
the site for nutrient loading (sugars and amino acids) for delivery
to developing tissues; these two regions of the plant are referred
to as source and sink tissues, respectively. Diffusion of nutrients
from the phloem CC–SE
complex into the surrounding phloem parenchyma cells & beyond
can be regulated by modulation of the PD size exclusion limit (i.e.,
the size of molecules that can pass through the PD microchannels
by simple diffusion). In source and long-distance phloem, NCAP
exchange across the boundary from CCs to the phloem parenchyma
most likely occurs through the selective (S)-NCAP pathway. In sink
tissues, the S-NCAP pathway appears to function in delivery of
NCAPs and RNA to specific tissues (see Sink tissues, middle image).
Localized unloading of NCAPs, through the GO pathway, could function
to remove errant molecules for degradation |
|
Long-Distance Signaling Systems Integrate
Whole-Plant Functions |
|
The unique properties
of PD for the exchange of metabolites and non-cell-autonomous
proteins (NCAPs) allow the plant to create specific symplasmic
domains within the phloem that permits both cell-to-cell and
long-distance delivery of information molecules. Input signals
that are perceived by mature leaves are transmitted into the
vascular system through the symplasm. Phloem-directed inputs
generate specific signaling molecules in response to environmental
conditions (see a , light, circadian rhythms, temperature,
mineral nutrient and water availability, etc.) or pathogen challenge
( b, virus, bacteria, fungi and insects). These signals enter
the phloem for long-distance trafficking through the petiole
( c ) and stem ( d ) and delivery to distant
organs such as the shoot apical meristem ( e ),
axillary meristems (f ) or root tissues
( g and h ).
This long-distance signaling system of the phloem affords
control over such processes as morphological traits on developing
leaves, partitioning of nutrients (sugars and amino acids), adaptive
responses to environmental inputs and resistance to pathogen
challenge. In this way,the PD and the phloem serve to inform
distantly located tissues and organs as to the current status
of factors that are likely to affect the livelihood of the
plant. Signals from the root system (mineral nutrient and
water availability, and so on) can move to the vegetative
regions of the plant through the xylem. |
|
A MYRIAD OF QUESTIONS STILL
REMAIN
CONCERNING THE NCAP PATHWAY |
|
|
To see more about individual research projects, please
select from the list below
|
Jeri Brandom |
Tien-Sin Yu |
Nien-Chen Huang |
Zaifeng Fan |
Eriko Miura |
Ya-Ling Lin |
Ken Toaka |
Cindy Huang |
|